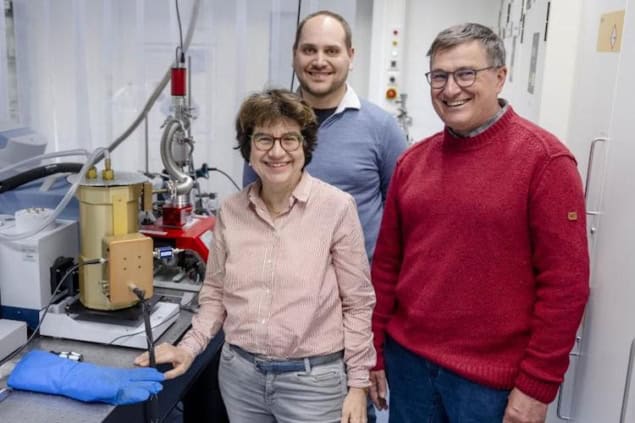
Contrary to some theorists’ expectations, water does not form hydrogen bonds in its supercritical phase. This finding, which is based on terahertz spectroscopy measurements and simulations by researchers at Ruhr University Bochum, Germany, puts to rest a long-standing controversy and could help us better understand the chemical processes that occur near deep-sea vents.
Water is unusual. Unlike most other materials, it is denser as a liquid than it is as the ice that forms when it freezes. It also expands rather than contracting when it cools; becomes less viscous when compressed; and exists in no fewer than 17 different crystalline phases.
Another unusual property is that at high temperatures and pressures – above 374 °C and 221 bars – water mostly exists as a supercritical fluid, meaning it shares some properties with both gases and liquids. Though such extreme conditions are rare on the Earth’s surface (at least outside a laboratory), they are typical for the planet’s crust and mantle. They are also present in so-called black smokers, which are hydrothermal vents that exist on the seabed in certain geologically active locations. Understanding supercritical water is therefore important for understanding the geochemical processes that occur in such conditions, including the genesis of gold ore.
Supercritical water also shows promise as an environmentally friendly solvent for industrial processes such as catalysis, and even as a mediator in nuclear power plants. Before any such applications see the light of day, however, researchers need to better understand the structure of water’s supercritical phase.
Probing the hydrogen bonding between molecules
At ambient conditions, the tetrahedrally-arranged hydrogen bonds (H-bonds) in liquid water produce a three-dimensional H-bonded network. Many of water’s unusual properties stem from this network, but as it approaches its supercritical point, its structure changes.
Previous studies of this change have produced results that were contradictory or unclear at best. While some pointed to the existence of distorted H-bonds, others identified heterogeneous structures involving rigid H-bonded dimers or, more generally, small clusters of tetrahedrally-bonded water surrounded by nonbonded gas-like molecules.
To resolve this mystery, an experimental team led by Gerhard Schwaab and Martina Havenith, together with Philipp Schienbein and Dominik Marx, investigated how water absorbs light in the far infrared/terahertz (THz) range of the spectrum. They performed their experiments and simulations at temperatures of 20° to 400°C and pressures from 1 bar up to 240 bars. In this way, they were able to investigate the hydrogen bonding between molecules in samples of water that were entering the supercritical state and samples that were already in it.
Diamond and gold cell
Because supercritical water is highly corrosive, the researchers carried out their experiments in a specially-designed cell made from diamond and gold. By comparing their experimental data with the results of extensive ab initio simulations that probed different parts of water’s high-temperature phase diagram, they obtained a molecular picture of what was happening.
The researchers found that the terahertz spectrum of water in its supercritical phase was practically identical to that of hot gaseous water vapour. This, they say, proves that supercritical water is different from both liquid water at ambient conditions and water in a low-temperature gas phase where clusters of molecules form directional hydrogen bonds. No such molecular clusters appear in supercritical water, they note.

Nanoconfined water enters intermediate solid-liquid phase
The team’s ab initio molecular dynamics simulations also revealed that two water molecules in the supercritical phase remain close to each other for a very limited time – much shorter than the typical lifetime of hydrogen bonds in liquid water – before distancing themselves. What is more, the bonds between hydrogen and oxygen atoms in supercritical water do not have a preferred orientation. Instead, they are permanently and randomly rotating. “This is completely different to the hydrogen bonds that connect the water molecules in liquid water at ambient conditions, which do have a persisting preferred orientation,” Havenith says.
Now that they have identified a clear spectroscopic fingerprint for supercritical water, the researchers want to study how solutes affect the solvation properties of this substance. They anticipate that the results from this work, which is published in Science Advances, will enable them to characterize the properties of supercritical water for use as a “green” solvent.